3.2.4.4. Reaction rate assays in pFI batch format: Catalytic determination of manganese and enzymatic assays
J. Ruzicka, M. Davis, M. Hatta and Ch. I. Measures © July 2024
In this section we introduce, for the first time, the reaction rate (RR) method in pFI batch format. While majority of flow-based assays are based on near equilibrium conditions, there are two reasons why an assay must be based on the initial reaction rate measurement:
- When reaction rate is too slow or
- When specific activity of a catalyst is to be determined
We will discuss the first case on catalytic determination of traces of manganese by Malachite Green spectrophotometry and the second case on enzymatic assays of substrates and catalytic activity of enzymes.
Introduction
To reach high sensitivity and acceptable sampling frequency, chromogenic assays are based on chemical reactions that reach equilibria within less than 2 minutes. This is achieved by optimizing pH, reagent composition, and by elevating temperature. Reaction rates of acid/base reactions and of complex formations are very fast, while rates of redox reactions are slower and therefore need to be accelerated by addition of catalyst. Example of the former is reaction of Fe(II) by ferrozine where equilibrium is reached within seconds. Example of the latter is reduction of molybdenum yellow to molybdenum blue by ascorbic acid that takes almost a minute. The result is that assay protocol can be designed to collect calibration and analysis data at near equilibrium or equilibrium conditions at sufficiently high sampling rates. However, this is not always feasible when the reaction rates are slow, even when accelerated by a catalyst. This is e.g., the group of enzymatic reactions such as oxidation of glucose by glucose oxidase, which therefore are based on reaction rate measurement. pFI in batch format offers several advantages for automation of RR assays because it performs measurement on homogenous well defined concentrations of reactants at stop flow conditions. This will eliminate artefacts unavoidable with CFA or FIA, where monitored solution moves through detector flow cell, where composition of reacting mixture, although in a very well reproduced gradient, is difficult to know.
Malachite Green Spectrophotometry
Manganese (III) catalyzes oxidation of the leukoform of redox dyes into intensely colored product. Thus, while Malachite Green in solution remains colorless, even in the presence of oxidant, it rapidly turns into a blue/green color presence of catalyst such as Mn or Fe.
Reaction rate of oxidation of MA Green catalyzed by Mn (III) is pH dependent and maximized in narrow pH range (4.1 to 4.6) while being also influenced by temperature, concentration of KIO3 and nitriloacetic acid (NTA).
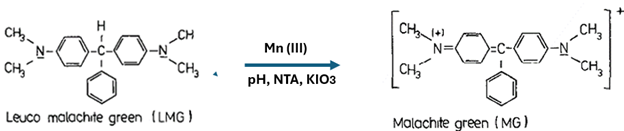
Automated in Flow Injection format (H, G) (Bruland, Feng), the three reagent assay protocol uses sequence of merging Mn sample with NH4 acetate buffer, followed by KIO3 solution, followed by slightly acidified MA Green solution.
In pFI format, two reagent assay protocol (A), sample is merged with acetate buffer containing KIO3 and NTA in the second step and merged with MA Green solution in the 3rd step. Holding coils are thermostated at 50 C and formation of MA Green is promoted by extending incubation time by stopping flow in the holding coil (10 sec) and in detector flow cell (40 sec) while reaction rate is monitored. Since sample zone is during steps 2 and 3 diluted 5+1 first with buffered reagents and next with Malachite Green, it becomes in the flow cell 30% diluted. The total incubation time is 50 seconds (10 sec in HC1 step 3 and 40 sec in FC step 4). Further details of the flow protocol (A) and the software protocol, along with optimized composition of used reagents and instrument description, are in the Appendix.
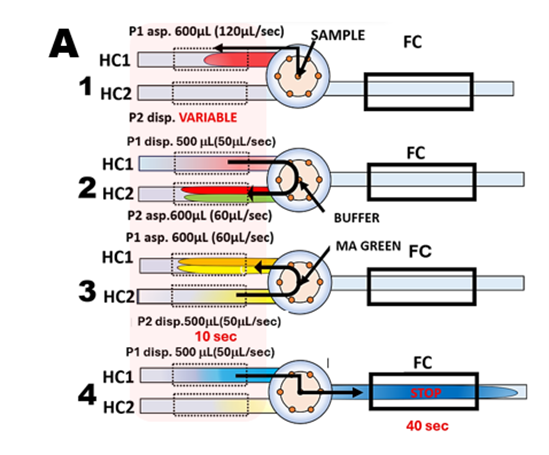
Preliminary experiments. To optimize the flow and software protocol the following items were investigated:
- Reaction rate of oxidation of Malachite Green (B)
- Influence of temperature on oxidation of MG (C)
- Positioning of reaction mixture in the detector flow cell (D)
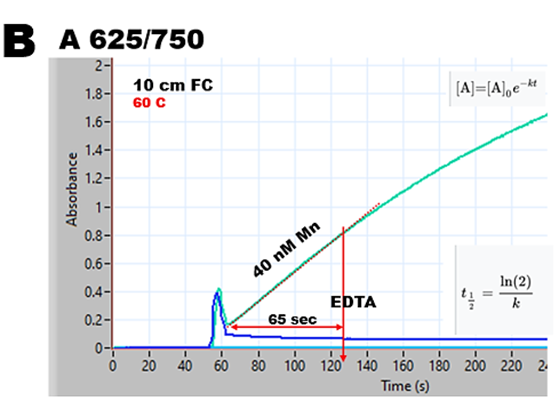
The initial, linear part of RR curve corresponds to t 1/2 of 65 seconds. If the reaction rate follows first order equation, the equilibrium will be reached (yield >95%) at 5 × 65 s = 325 seconds which, combined with 60 seconds initial time yields sampling frequency 10 s/hr, which makes measurement at equilibrium impractical. However, by limiting stop flow period in FC to 40 seconds and thus total time to 110 seconds (C), the sampling frequency will increase to 33 s/hr. Thus sensitivity of determination and sampling frequency are related. The dark blue line was recorded when 40 nM Mn sample was replaced by 0.001 M EDTA solution which binds Mn (III), thus preventing oxidation of MG. This observation confirms that at optimized conditions and absence of Mn MG is not oxidized and reagent blank is stabilized. The light blue line was recorded when flow cell was filled with DI water, confirming time stability of spectrophotometry. Therefore the difference between absorbance values of dark and light blue tracks can be used to calculate limit of detection (LOD) of the method, in absence of manganese in the reagent.
Reaction rate of endothermic reactions increases with increasing temperature and oxidation of MG follows this law (C).
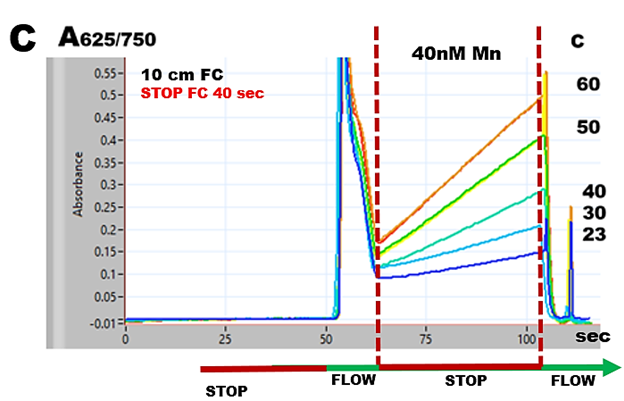
By using 40 second stop flow in flow cell, a series of 40 nM Mn samples were analyzed while holding coils were thermostated at increasing temperatures. 50 C was selected for the following experiments since there is only a small gain of RR at 60 C and also because use of temperature above 50 C for sea water analysis may cause precipitation.
The position of reacting mixture within the flow cell is critical in pF batch mode (3.2.3.). It is the transport volume of forward flow from HC1 to flow cell (step 4) that must be optimized to operate in batch mode, and to maximize sensitivity by monitoring most concentrated, homogenous section of reacting mixture arrested in the flow cell. This was investigated by using 40 nM Mn sample, 40 seconds stop flow time at 50 C, by recording spectra and reaction rate curves (D).
.png.aspx?width=800&height=373)
As the reaction mixture advances into the flow cell, which integrates absorbance within its volume (50 μL), the spectra (recorded at the end of stop flow period) as well as the slope of RR responses change. This is because at 200 μL transport volume, FC is mostly filled by front boundary between DI (carrier) and reacting mixture, comprising reacting mixture of higher ionic strength. The resulting refractive index forms a lens that deflects light beam away from detector resulting in high absorbance response within which MG spectrum is buried. With increasing transport volumes MG spectrum gradually emerges and it becomes maximized at 500 μL. It decreases as the reacting mixture becomes diluted (by carrier) at larger transport volumes.
Calibration and sensitivity of RR determination was investigated by using 20 nM Mn standard serially diluted by auto calibration program (3.2.5.2.), 120 sec stop flow period, temperature at 50 C and optimized reagent composition (Appendix) with mixing volumes shown in the flow chart (A). Spectra recorded at the end of stop flow period (left) and corresponding calibration runs (right) are shown below (E).
.png.aspx?width=800&height=361)
When autocalibration run was completed, 0.001M EDTA sample as analyzed to obtain a recording of reagents mixture in absence of Mn (III) that was in this way removed by complexation. Thus, the arow shows the difference between reagent baseline and reagent BLANK due to manganese impurities in the reagents.
.png.aspx)
The calibration graph (F) generated by software is based on the maximum absorbance value captured within data collection window (WIN) and baseline value (BS) recoded while flow cell was filled with DI water (carrier). The calibration in the range of 0 to 15 nM Mn is linear with slope of 0.034 A/nM Mn. This yields the method LOD = 0.13 nM Mn, based on difference between reagent baseline (EDTA) and zero absorbance (BS), while difference between BLANK and BS yields reagent based LOD = 0.75 nM Mn, which is due to reagent contamination by manganese, equal to 4.7 nM, found from difference between BLANK and EDTA absorbancies.
Catalytic determination of manganese by MG spectrophotometry on continuous flow basis, with (H) and without (G) on-column preconcentration has been used for determination of Mn in sea water with sampling rate 10 s/hr (G) and 6 s/hr (H). The RR method presented here is sufficiently sensitive to cover the concentrations of TD Mn in sea water with stop flow period of 80 seconds (slope 0.025 A/nM Fig. E right), and sampling frequency 24 s/hr. By increasing length of the flow cell from present 10 cm up to 40 cm (LLP FC), sensitivity of determination or sampling frequency can be further increased. While this confirms potential of this method for determination of traces of manganese in sea water, several issues need to resolved:
- Sampling frequency shall be adjusted to desired sensitivity
- LOD must be maintained below 1 nM Mn by reducing BLANK, by careful purification of reagents and DI water
- The influence of salinity on reaction rate must be investigated
- The method must be verified by analysis of reference materials
Enzymatic assays
Enzymatic assays fall into two categories: determination of substrate by monitoring its rate of decomposition, catalyzed by enzyme, or determination of enzyme activity by measuring rate of decomposition of substrate. In both cases initial reaction rate is measured at (pseudo) first order kinetics (B), which are maintained by using high enzymatic activity for substrate assay and high substrate concentration for determination of activity of enzyme.
According to Hansen’s FIA Database only 1% of published works is dealing with enzymatic determination of substrates and none are listed for determination of enzymatic activity, This is, because concentration gradient of reacting mixture within the flow cell makes determination of substrate difficult to optimize, and determination of enzymatic activity impossible. This is explained on example of determination of glucose by conversion to gluconic acid catalyzed by glucose oxidase monitored by spectrophotometry which was performed in Sequential Injection mode (K) (3.1.1.)
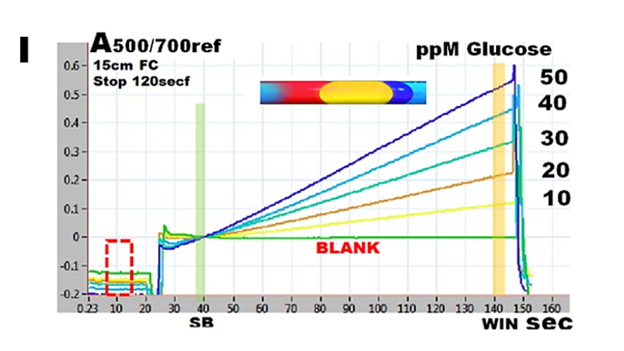
The initial reaction rates at all glucose concentrations and resulting calibrations are linear and well reproduced, (2.2.11.B) because in SI mode the concentration gradient formed within “sandwich” of reagent/sample/reagent sequence is well reproduced. However, the exact concentrations of reactants within the flow cell are unknown. Therefore while this does not impair determination of substrate, which is supported by calibration with standard solutions, determination of activity of glucose oxidase by Sequential Injection is not possible.
This is because enzymatic activity is calculated by
a = nt = r × V where a is enzyme activity nt are moles of substrate converted per unit of time r is rate of reaction V is reaction volume. The enzyme unit (U) = 1 μM/min.
However, in pFI batch mode concentration of substrate in flow cell can be calculated from dilution ratios (A) and reaction volume, which is flow cell volume (50 μL for 10 cm) that holds homogenously mixed uniform concentration of reactants (3.2.3.) NOTE; Dilution ratios for manganese determination were chosen to maximize sensitivity of determination by minimizing sample dilution (500 + 100). For enzymatic assays dilution 300+300 will improve reproducibility of determinations.
We hope that use of pF in batch mode (3.2.4.3.) will find application in the wide range of enzymatic assays, which are presently automated by discrete batch type analyzers.
Appendix
Reagents
Buffered Reagent 0.4% NTA (nitrilotriacetic acid), 0.2% KIO3 in 0.25 M Ammonium Acetate buffer pH 4.3.
Buffered Reagent preparation. Since 0.4% NTA is approx. 0.02M, it must be neutralized by NH4OH. Therefore, place 50 mL DI in transparent container, dissolve 400 mg NTA, add and dissolve 200 mg KIO3, and add 5 mL of 1M NH4OH. Add 25 mL of 2M Ammonium Acetate buffer pH 4.3 and 25 mL DI. Check pH and adjust by further addition of NH4OH to pH 4.3 to 4.6. Use pH meter. NOTE: below pH 4, reaction rate will decrease. Above pH 5, MG will be oxidized.
Collect effluent from flow cell and check pH. This is important if SW samples were acidified.
Malachite Green (MG): 0.01 g / 100 mL in 0.015 M HCl
Mn Stock Solution: prepared from commercial standard (1000 ppm), which is in 2% HNO3 solution, by diluting to 100 μM with DI water
Mn Standard Solution: 40nM Mn or 20nM Mn was prepared from stock solution by diluting with DI water
Instrument
Multipurpose pFI instrument configured as shown in Tutorial 3.2.7.
The first wait time is in holding coil, the second is in flow cell. Blue box is transport volume into flow cell.